Carbon dioxide and diving
Basic carbon dioxide physiology for divers with Associate Professor Simon Mitchell, University of Auckland.
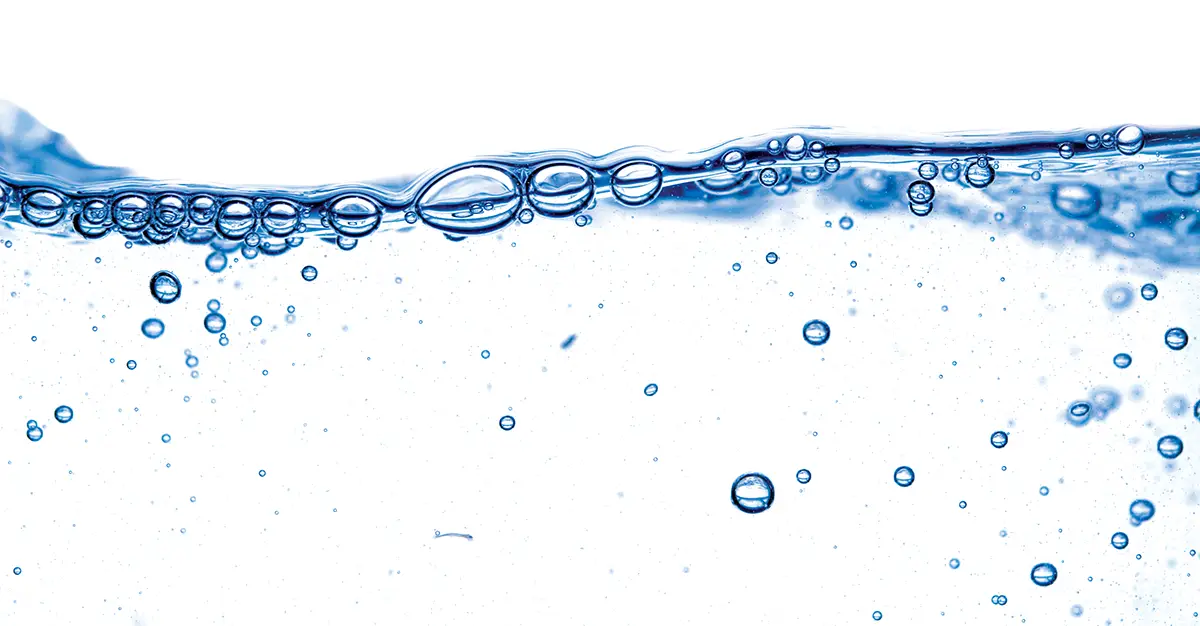
This is the first in a series of articles that will appear in Dive New Zealand with the aim of enhancing knowledge of selected practically important issues in diving physiology and medicine.
These articles are intended for divers who want a little more detail on the subjects than is usually available in diving courses or course materials. This could be characterised as ‘advanced knowledge’, but I hope to present the information in clear and understandable way.
The theme of the first series will be carbon dioxide (CO2), and in this first article I will explain what CO2 is and why it is important in diving. In two or three subsequent articles I will describe some fascinating research we have been conducting here in New Zealand to answer important related questions. Some of this work has been made possible by local divers volunteering as subjects, and the least we can do in return is to feed the resulting knowledge back into the community.
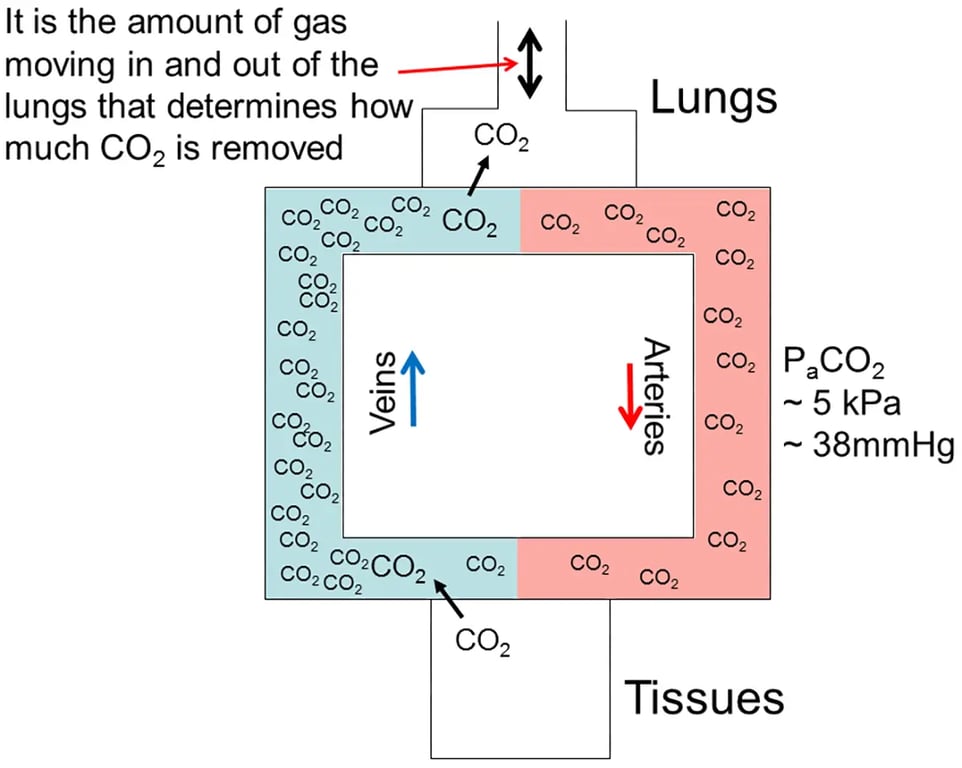
What is CO2?
Most divers will be aware that the production of energy to drive virtually all biological processes in the body requires oxygen. When oxygen is used in the various body tissues for energy metabolism another gas, CO2, is produced. Its rate of production is directly proportional to oxygen consumption. In some conditions, such as during exercise when large amounts of oxygen are consumed by working muscle, more CO2 is produced, whereas at rest when less oxygen is consumed, less CO2 is produced. CO2 is a gas, but when produced in the body it is initially in dissolved form. It diffuses through tissue fluids into the blood and is carried back to the lungs in the veins (see Figure 1). At the lungs CO2 diffuses into the alveoli (the lung’s tiny air sacs) and in doing so it becomes a gas again. When gas is exhaled from the lungs then CO2 is eliminated. Not all the CO2 is removed as the venous blood passes through the lungs, and indeed, it is the pressure of CO2 in the arterial blood that is monitored in control of breathing (see Figure 1.).
Control of CO2 levels in the body
This process of CO2 elimination is dependent on there being a pressure gradient for diffusion of CO2 from the tissues to venous blood, and from venous blood into the lung alveoli. Put simply, the pressure of CO2 is higher in the tissues than the blood, and higher in the blood than the alveoli so molecules of CO2 diffuse in the direction of this pressure gradient. The gradient is maintained by removal of CO2 from the alveoli by breathing. This is a key point. The more gas you breathe in and out of the lungs, the more CO2 you eliminate, and vice versa.
In other words, if you take a series of rapid deep breaths you can increase elimination of CO2, and this so-called ‘hyper- ventilation’ is what some free divers (unwisely) do to lower their blood CO2 prior to a long breath-hold. Equally, if you take shallow breaths, breathe slowly, or even hold your breath you decrease elimination of CO2 and it will accumulate in the blood.
Under normal circumstances the pressure of CO2 dissolved in tissues and blood is carefully and automatically regulated by the body. The brain has what is effectively a CO2 sensor that indirectly monitors blood levels, and adjusts breathing accordingly. Thus, if the blood CO2 starts to rise, then your brain will drive you breathe more (either by increasing your breathing rate or breath size or both), and if your blood CO2 starts to fall, then your brain will cause you to breathe less so that CO2 levels rise again. All of this is happening at a completely subconscious level as you sit reading this. In most people the brain is ‘set’ to maintain a dissolved PCO2 of about 5 kilopascals (kPa) (or 0.05 ATA or 38mmHg depending on what units you prefer to use) in the arterial blood.
Disruption of CO2 control
This control system is imperfect and under some circumstances it can become less precise. For example, if the work involved in breathing increases unnaturally (which occurs in diving for a variety of reasons) the breathing controller in the brain appears predisposed to allowing the blood CO2 to rise rather than drive the extra work required to move sufficient gas in and out of the lungs to keep CO2 at normal levels. To conceptualise this, think of circulatory system in Figure 1 as a conveyor belt, going round and round in a clockwise direction.
Think of the tissues on one side as a person putting objects (in this case CO2 molecules) on the conveyor belt, and the lungs on the other side as a person taking objects off the conveyor belt. If the person taking objects off the conveyor works more slowly than the person putting them on, then object numbers on the conveyor will start to rise. When this happens with CO2 we refer to it as ‘CO2 retention’; a situation in which we are not breathing enough to eliminate the CO2 we are producing. The more the work of breathing increases (eg. because we are deeper breathing a denser gas, or because our regulator is performing poorly), and the more CO2 that is being produced (eg. because of exercise) then the more likely CO2 retention is to occur.
Interestingly, there is also significant variability between individuals in respect of their tendency to retain CO2.
Some peoples’ respiratory controller will adjust breathing to maintain their normal level of CO2 irrespective of how much the work of breathing increases, whilst others are very vulnerable to CO2 retention when an increase in work of breathing blunts the sensitivity of normal control. The latter group are often referred to as ‘CO2 retainers’. It is simply a failure to breathe sufficiently to eliminate all the CO2 that is being produced in the body. This can occur any time the work of breathing increases, and in diving this applies to normal open circuit scuba diving and also closed circuit rebreather diving. It is important to understand, however, that the discussion to this point has had nothing to do with CO2 breaking through a CO2 scrubber and consequent CO2 rebreathing when using a rebreather. CO2 rebreathing certainly can occur if the scrubber fails during use of a rebreather, and this is a second cause for developing high CO2 levels in diving, but it is separate to the mechanisms I have discussed so far. CO2 rebreathing will be discussed further in the second article in this series.
Why high levels of CO2 are bad in diving
Rising blood CO2 (‘hypercapnia’) is a problem in diving for several reasons. First it can cause unpleasant symptoms such as headache, anxiety and shortness of breath. These can precipitate panic. If the levels get high enough CO2 can cause incapacitation and unconsciousness. Collectively, these manifestations are often referred to as ‘CO2 toxicity’. Interestingly, those who tend to retain CO2 generally suffer fewer early unpleasant symptoms, and indeed, may not develop symptoms until they are close to the second tier of problems (incapacitation and unconsciousness). To give you some sense of the small changes in arterial blood levels required for these phenomena, around 5kPa is the average normal level, 6.2kPa is the upper limit of the normal range, and over 8.5kPa sudden incapacitation is likely. Experiments show that levels between 6.5 and 7.5 are not uncommon in divers working underwater. The point is that small changes in PCO2 of 1kPa or less can have very important implications for the safety of the diver.
The second reason high levels of CO2 are a problem in diving is that CO2 can precipitate other diving related problems. In particular, CO2 is a narcotic gas and high CO2 levels will substantially worsen nitrogen narcosis. Similarly, high levels of CO2 are known to substantially increase the risk of cerebral oxygen toxicity which can manifest as a seizure. The mechanism for this is probably that CO2 causes a substantial increase in blood flow to the brain, thus increasing the brain’s exposure to oxygen when a diver is inspiring a high pressure of oxygen. It goes without saying that the loss of consciousness associated with a seizure would often be fatal under water. This is unlikely to be a hazard in scuba air diving, but it is an issue that technical divers must consider because they often breathe oxygen at high pressures for extended periods, and thus are exposed to a risk of oxygen toxicity. It follows that technical divers in particular need to be very careful to avoid hypercapnia when their oxygen exposures are high.
Future articles in this series
In the next article I will describe a recent study in which we investigated technical divers for CO2 retention at the end of long decompressions when their oxygen exposures were high and their risk of oxygen toxicity was therefore maximal. In future articles I will discuss issues like the effectiveness of the five-minute pre-breathe on a rebreather for detecting CO2 scrubber problems prior to diving, which CO2 absorbent is best, and how CO2 scrubbers are best stored between dives if they are not discarded.